
Stars are born in clouds of gas and dust, making it difficult to observe their early development. But researchers at Chalmers have now succeeded in simulating how a star with the mass of the sun absorbs material from the surrounding disk of material—a process called accretion.
The researchers simulated four stars with the same mass but with very different magnetic fields to see how that affected the accretion. The study is published in Astronomy & Astrophysics.
“These are some of the first high-resolution simulations where we explore the middle stage of protostar evolution where most of the star’s mass is gathered, and which haven’t been studied in this much detail before. Our four simulations cover two months each in the life of a protostar and they took a computational effort equivalent to about 2 million CPU hours to perform,” says Brandt Gaches, astronomer at Chalmers.
One of many unanswered questions in astronomy is how newborn stars—protostars—accrete mass from their surrounding clouds of gas. While new and updated telescopes have increased their observational capabilities, theoretical models of the process have been lacking. To explore the early days—or the early hundreds of thousands of years to be more correct—of star formation, Brandt Gaches and colleagues decided to create detailed simulations.
“The main, fundamental question was: How do early protostars accrete their mass? There have been a few models proposed for different types of protostars, but we wanted to start from scratch and ask: For a protostar with similar mass to the sun, how would different magnetic field strengths affect the accretion process? Does it fundamentally change things, or is the difference marginal?” says Gaches, a Cosmic Origins Fellow at Chalmers University of Technology.
Focus on magnetic fields
The magnetic field can vary a lot depending on which material the star has gathered from the surrounding clouds. The surface magnetic field strength of young protostars is not well constrained, and when and how they gain their magnetic fields is not well known. However, their magnetic fields should play a significant role in how they grow their mass and interact with their natal environment.
To get a closer look at how the magnetic fields impact the accretion rate, the researchers modeled four protostars, with the mass and gravity like our sun, but where the magnetic fields range from 10 Gauss—also roughly the same as our sun—to 2,000 Gauss. The goal was to see if and how the magnetic fields influenced how the star accretes material from the surrounding disk.
“In the 10 Gauss case, where the protostar is weakly magnetized, we can see that the gas flows freely from the disk directly to the surface of the protostar. We can also observe that a lot of material is ejected from the star’s poles.
“In the highly magnetized case (2,000 Gauss), the protostar’s magnetic field is strong enough to cut off the disk, stopping the direct gas flow. Instead, gas only flows along the magnetic field lines that connect the disk to the protostar, in areas closer to the poles. The matter prefers to follow the field lines, creating a much more defined flow—with virtually no material escaping from the poles,” says Gaches.
But even if the process looks a lot different in the two extremes, there is not a huge difference in the amount of material that the stars accrete—the difference instead lies in how the material accretes over the two months of simulations.
“In the 10 Gauss case, the accretion rate is highly variable, fluctuating at a rate by factors of up to 10 over hourly timescales. This is because material is essentially raining down chaotically,” says Gaches.
As the magnetic fields increase in the 500 and 1,000 Gauss simulations, the gas flows become more stable, as the magnetic field helps organize the flow and reduce variability. But at 2,000 Gauss, the variability reappears, in a very different way. Most of the material flow along the field lines towards the star’s poles, in an orderly fashion.
But now and then the simulations also show a build-up of material that crashes directly into the star in sudden and irregular bursts. This is due to gas piling up at the magnetic boundary and eventually pushes through, creating the bursts of accretion.
“We expected a more continuous flow of material along the magnetic field lines, so these spikes in the accretion rate was unexpected,” says Brandt.
“The simulations also showed, surprisingly, that regardless of the mode of accretion, the fraction of the surface of the protostar onto which the majority of the gas falls does not change much on average.”
More work to come on the physics on star formation
“We think these simulations motivate further observations of magnetic fields in protostars, and that our simulations can be of great help when interpreting such observations of the accretion process.”
The simulations will be extended in future work for a broader look at the physics of star formation. The high resolution and long timeline make it able to explore a wide range of interesting protostellar physics.
“One of the key goals of these simulations was to generate a large dataset to explore the physics of star formation. Each model has around 3,000 single images spread out over a time period of 60 days. This gives us high time and spatial resolution, allowing us to piece together detailed information.” https://phys.org/news/2025-01-high-resolution-simulations-explore-physics.html
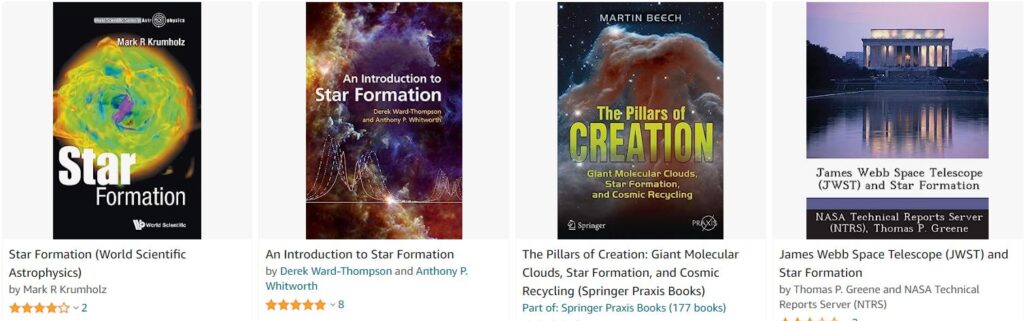
Recent Comments