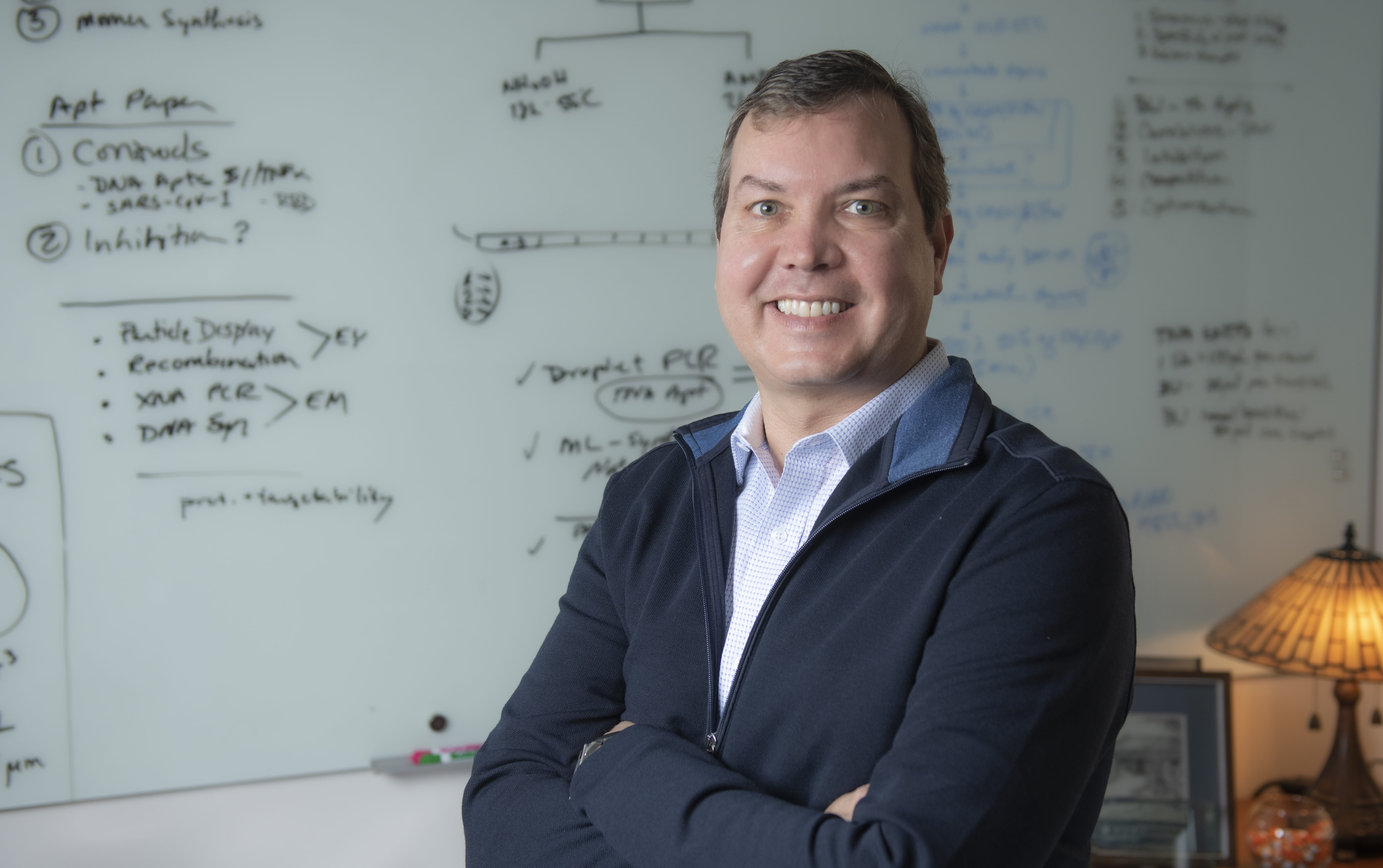
Discovery advances development of new therapeutic options for cancer and other diseases. A research team led by the University of California, Irvine has engineered an efficient new enzyme that can produce a synthetic genetic material called threose nucleic acid. The ability to synthesize artificial chains of TNA, which is inherently more stable than DNA, advances the discovery of potentially more powerful, precise therapeutic options to treat cancer and autoimmune, metabolic and infectious diseases.
Read More
Recent Comments