
In 1960, while preparing for the first meeting on the Search for Extraterrestrial Intelligence (SETI), legendary astronomer and SETI pioneer Dr. Frank Drake unveiled his probabilistic equation for estimating the number of possible civilizations in our galaxy—aka The Drake Equation. A key parameter in this equation was ne, the number of planets in our galaxy capable of supporting life—aka “habitable.” At the time, astronomers were not yet certain other stars had systems of planets. But thanks to missions like Kepler, 5,523 exoplanets have been confirmed, and another 9,867 await confirmation.
Based on this data, astronomers have produced various estimates for the number of habitable planets in our galaxy—at least 100 billion, according to one estimate. In a recent study posted to the arXiv preprint server, Professor Piero Madau introduced a mathematical framework for calculating the population of habitable planets within 100 parsecs (326 light-years) of our sun. Assuming Earth and the solar system are representative of the norm, Madau calculated that this volume of space could contain as much as 11,000 Earth-sized terrestrial (aka rocky) exoplanets that orbit within their stars’ habitable zones (HZs).
Prof. Madau is a professor of astronomy and astrophysics at the University of California, Santa Cruz (UCSC). Central to his study is the Copernican Principle, named for famed Polish astronomer Nicolaus Copernicus, inventor of the heliocentric model. Also known as the Cosmological Principle (or Mediocrity Principle), the principle states that neither humans nor Earth are in a privileged position to observe the universe. In short, what we see when we look upon the solar system and out into the cosmos is representative of the whole.
For his study, Madau considered how time-dependent factors have played a vital role in the emergence of life in our universe. This includes the star formation history of our galaxy, the enrichment of the interstellar medium (ISM) by heavy elements (forged in the interior of the first population of stars), the formation of planets, and the distribution of water and organic molecules between planets. As Madau explained to Universe Today, the central role of time and age are not explicitly stressed in the Drake Equation:
“The Drake equation amounts to a useful pedagogical summary of the factors (probabilities) that may affect the likelihood of detecting life-bearing worlds—and eventually technologically advanced extraterrestrial civilizations—around us today. But that likelihood and those factors depend, among other quantities, on the star formation and chemical enrichment history of the local Galactic disk, as well as on the timeline of the emergence of simple microbial and eventually complex life.”
Earth is a relative newcomer to our galaxy, having formed with our sun roughly 4.5 billion years ago (making it less than 33% the age of the universe). Life, meanwhile, took about 500 million years to emerge from the primordial conditions that existed on Earth ca. 4 billion years ago. About 500 million years after that, photosynthesis emerged in the form of single-celled organisms that metabolized carbon dioxide and produced oxygen gas as a byproduct. This gradually altered the chemical makeup of our atmosphere, triggering the Great Oxidation Event about 2.4 billion years ago and the eventual rise of complex life forms.
A long and complex process of chemical and biological evolution followed, eventually leading to conditions suitable for complex life and the emergence of all known species. Given the importance of these time-dependent steps, Madau argues that the Drake Equation is only part of the story. Looking beyond it, he created a mathematical framework to estimate when “temperate terrestrial planets” (TTPs) formed in our corner of the galaxy and microbial life could have emerged.
This framework allows astronomers to determine which potential target stars (based on mass, age, and metallicity) may be optimal candidates in the search for atmospheric biosignatures. As Madau described it, his approach consists of considering the local population of long-lived stars, exoplanets, and TTPs as a series of mathematical equations, which can be solved numerically as a function of time:
“These equations describe the changing rates of star, metal, giant, and rocky planets, and habitable world formation over the history of the solar neighborhood, the locale where more detailed calculations are justified by an avalanche of new data from space-based and ground-based facilities and the target of current and next-generation stellar and planetary surveys. The equations are statistical in nature, i.e. they do not describe the birth and evolution of individual planetary systems but rather the changing (over time) population (by number) of TTPs within 100 parsecs of the sun.”
Ultimately, Madau’s analysis showed that within 100 parsecs of the sun, there may be as many as 10,000 rocky planets orbiting with their star’s HZs. He also found that the formation of TTPs near our solar system was likely episodic, starting with a burst of star formation roughly 10–11 billion years ago, followed by another event that peaked about 5 billion years ago that produced the solar system. Another interesting takeaway from Madau’s mathematical framework indicates that most TTPs within 100 parsecs are likely older than the solar system, confirming that we are a relative latecomer to the party.
Equally interesting are the implications this study could have on the search for extraterrestrial life. Using the generally accepted timeline of the emergence of life on Earth (abiogenesis) and applying a conservative estimate of the prevalence of life on other planets—the fl parameter of the Drake Equation—Madau’s framework also indicated how far away the closest exoplanet harboring life could be:
“So, if microbial life arose as soon as it did on Earth in more than 1% of TTPs (and that is a big if), then one expects the closest, life-harboring Earth-like planet to be less than 20 pc away [65 light-years],” he said. “This may be cause for some cautious optimism in the search for habitability markers and biosignatures by the next generation of large ground-based facilities and instrumentation. Needless to say, biosignatures are going to be extremely challenging to detect. And it is also possible that life may be so rare that there are no biosignatures within a kpc or more for us to detect.”
Of course, there are no guarantees that any TTPs near our solar system could support life. The causes and commonality of abiogenesis is one of the least-understood scientific pursuits, mainly because it is so data-poor. Armed with only one example (Earth and terrestrial organisms), scientists cannot confidently say what combination of conditions is necessary for life to emerge. Madau also stresses that (like the Drake Equation), his approach is statistical in nature. Nevertheless, his work could have significant implications for astrobiology in the near future.
Using our solar system as a guide, along with many other parameters for which there are volumes of data (i.e., star formation, mass, size, metallicity, and the number of nearby exoplanets orbiting within a star’s HZ), scientists will be able to prioritize star systems for investigation using next-generation telescopes.
Said Madau, “The yield and characterization of Earth-like planets will be a primary science metric for future space-based flagship missions. With the fast-approaching opportunity to make a search for habitable environments and life on exoplanets comes the real challenge of actually designing an optimal observational strategy. Detailed spectral studies of a few exoplanet atmospheres must be accompanied by population studies designed to reveal trends in planet properties and statistical studies that will allow us to evaluate the likelihood of biosignature detectability.” https://phys.org/news/2023-09-extraterrestrial-life-light-years-earth-average.html
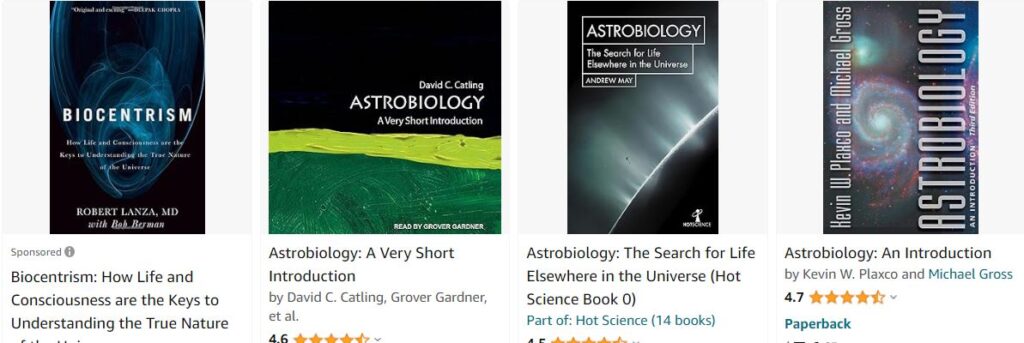
Recent Comments