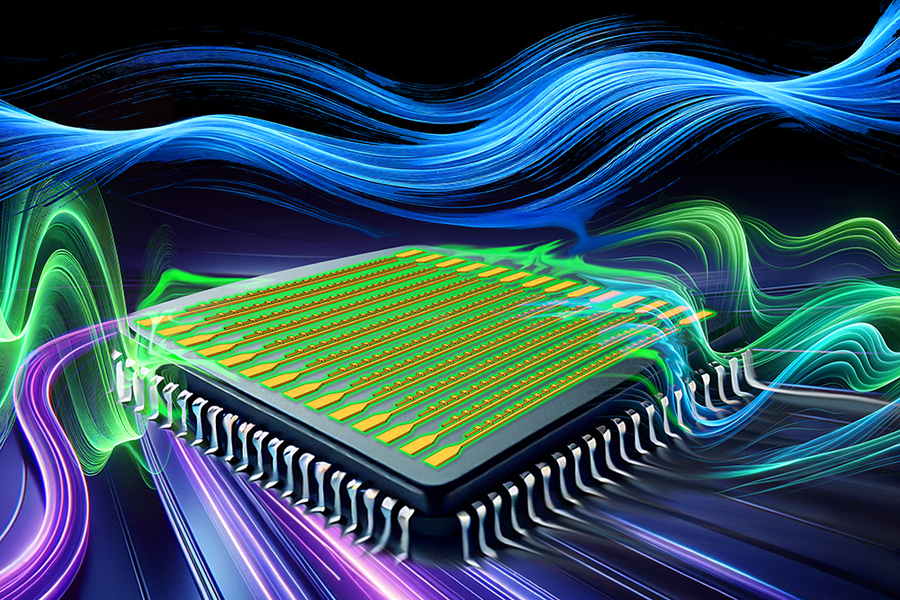
Credits:Image: Sampson Wilcox/Research Laboratory of Electronics
Imagine how a phone call works: Your voice is converted into electronic signals, shifted up to higher frequencies, transmitted over long distances, and then shifted back down so it can be heard clearly on the other end. The process enabling this shifting of signal frequencies is called frequency mixing, and it is essential for communication technologies like radio and Wi-Fi. Frequency mixers are vital components in many electronic devices and typically operate using frequencies that oscillate billions (GHz, gigahertz) to trillions (THz, terahertz) of times per second.
Now imagine a frequency mixer that works at a quadrillion (PHz, petahertz) times per second—up to a million times faster. This frequency range corresponds to the oscillations of the electric and magnetic fields that make up light waves.
Petahertz-frequency mixers would allow us to shift signals up to optical frequencies and then back down to more conventional electronic frequencies, enabling the transmission and processing of vastly larger amounts of information at many times higher speeds. This leap in speed isn’t just about doing things faster; it’s about enabling entirely new capabilities.
Lightwave electronics (or petahertz electronics) is an emerging field that aims to integrate optical and electronic systems at incredibly high speeds, leveraging the ultrafast oscillations of light fields. The key idea is to harness the electric field of light waves, which oscillate on sub-femtosecond (10-15 seconds) timescales, to directly drive electronic processes.
This allows for the processing and manipulation of information at speeds far beyond what is possible with current electronic technologies. In combination with other petahertz electronic circuitry, a petahertz electronic mixer would allow us to process and analyze vast amounts of information in real time and transfer larger amounts of data over the air at unprecedented speeds.
The MIT team’s demonstration of a lightwave-electronic mixer at petahertz-scale frequencies is a first step toward making communication technology faster, and progresses research toward developing new, miniaturized lightwave electronic circuitry capable of handling optical signals directly at the nanoscale.
In the 1970s, scientists began exploring ways to extend electronic frequency mixing into the terahertz range using diodes. While these early efforts showed promise, progress stalled for decades. Recently, however, advances in nanotechnology have reignited this area of research. Researchers discovered that tiny structures like nanometer-length-scale needle tips and plasmonic antennas could function similarly to those early diodes but at much higher frequencies.
A recent study published in Science Advances by Matthew Yeung, Lu-Ting Chou, Marco Turchetti, Felix Ritzkowsky, Karl K. Berggren, and Phillip D. Keathley at MIT has demonstrated a significant step forward. They developed an electronic frequency mixer for signal detection that operates beyond 0.350 PHz using tiny nanoantennae. These nanoantennae can mix different frequencies of light, enabling analysis of signals oscillating orders of magnitude faster than the fastest accessible to conventional electronics.
Such petahertz electronic devices could enable developments that ultimately revolutionize fields that require precise analysis of extremely fast optical signals, such as spectroscopy and imaging, where capturing femtosecond-scale dynamics is crucial (a femtosecond is one-millionth of one-billionth of a second).
The team’s study highlights the use of nanoantenna networks to create a broadband, on-chip electronic optical frequency mixer. This innovative approach allows for the accurate readout of optical wave forms spanning more than one octave of bandwidth. Importantly, this process worked using a commercial turnkey laser that can be purchased off the shelf, rather than a highly customized laser.
While optical frequency mixing is possible using nonlinear materials, the process is purely optical (that is, it converts light input to light output at a new frequency). Furthermore, the materials have to be many wavelengths in thickness, limiting the device size to the micrometer scale (a micrometer is one-millionth of a meter).
In contrast, the lightwave-electronic method demonstrated by the authors uses a light-driven tunneling mechanism that offers high nonlinearities for frequency mixing and direct electronic output using nanometer-scale devices (a nanometer is one-billionth of a meter).
While this study focused on characterizing light pulses of different frequencies, the researchers envision that similar devices will enable one to construct circuits using light waves. This device, with bandwidths spanning multiple octaves, could provide new ways to investigate ultrafast light-matter interactions, accelerating advancements in ultrafast source technologies.
This work not only pushes the boundaries of what is possible in optical signal processing but also bridges the gap between the fields of electronics and optics. By connecting these two important areas of research, this study paves the way for new technologies and applications in fields like spectroscopy, imaging, and communications, ultimately advancing our ability to explore and manipulate the ultrafast dynamics of light. https://news.mit.edu/2024/nanostructures-enable-chip-lightwave-electronic-frequency-mixer-0904
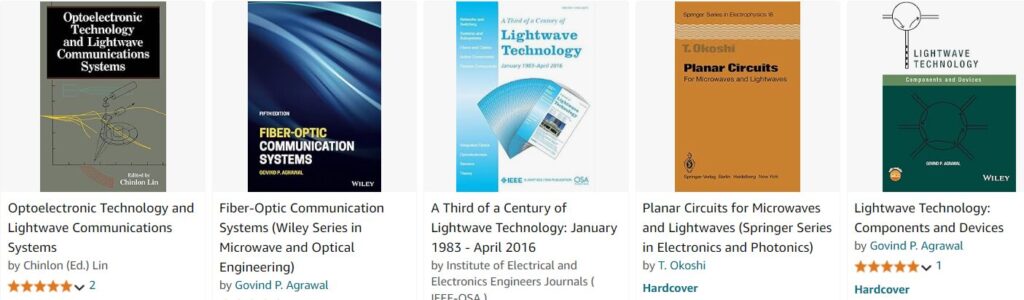
Recent Comments